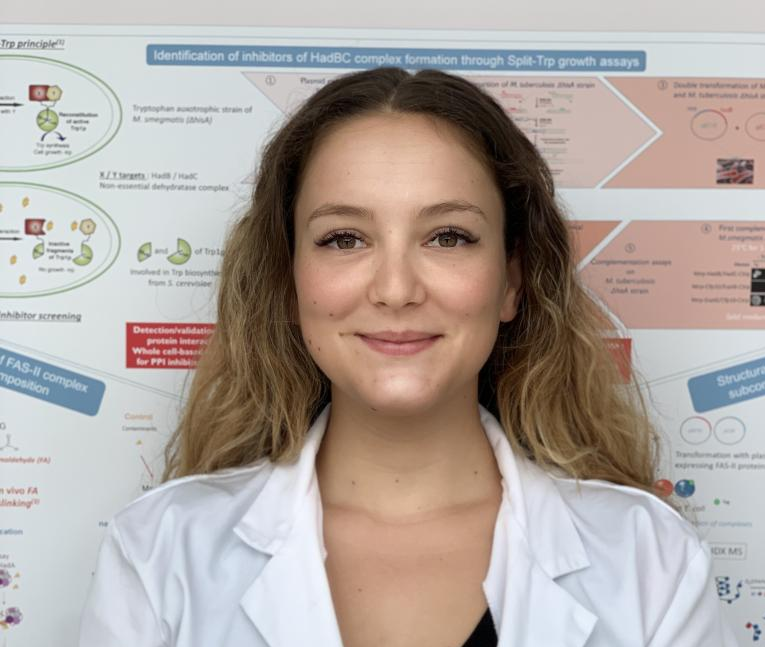
Pascaline Bories’s PhD defensis
In cellulo targeting of protein-protein interactions within Mycobacterium tuberculosis FAS-II complex: an innovating approach for new TB drugs
Responsible for more than 4,000 deaths per day worldwide, tuberculosis is the most killing infectious disease due to a single pathogen agent, Mycobacterium tuberculosis (Mtb). Due to the appearance of strains that are multiresistant to standard antibiotherapy, the development of antituberculosis drugs with new modes of action has become urgent. Drugs classically used for TB treatment are targeting active sites of individual enzymes essential for Mtb survival, leading to frequent apparition of resistances. This unconventional research project consists in targeting, directly on whole-cell bacteria, protein-protein interactions (PPI) that are non-essential for Mtb survival but involved in the bacillus virulence. This should allow us to identify molecules able to pass through the highly impermeable mycobacterial envelope, and to inhibit the pathogen growth within the infected host while limiting the pressure to develop resistances.
The Fatty Acid Synthase II (FAS-II) multienzyme system, playing a role in the biosynthesis of very important lipidic virulence factors of the tubercle bacillus, represents a pertinent and validated therapeutic target. This complex is already the target of several first line anti-TB drugs, such as isoniazid and ethionamide. FAS-II is made of monofunctional acyl carrier protein (ACP)-dependant enzymes achieving the 4 main reactions steps of mycolic acid chain elongation cycles. The third step, a dehydration reaction, is performed by (3R)-hydroxyacyl-AcpM dehydratases that assemble in heterodimers HadAB and HadBC. More than 20 years after the Mtb whole genome sequencing, the exhaustive composition of FAS-II and its precise function are not yet determined. However, a recent study in the team led to the discovery of a novel FAS-II partner enzyme, HadBD, which is not essential but bacillus virulence-liked.
The objective of the work performed during my thesis is to identify molecules able to disrupt PPIs within FAS-II complex that are not essential for Mtb survival, but involved in its virulence.
To do so, the 1st part of my project consisted in the caracterisation of the heterodimer HadBD, in collaboration with L. Mourey’s team (IPBS), thanks to in cellulo interactions analyses, enzymatic activity assays of mutated proteins and crystallographic studies.
The 2nd part of my thesis led to the development of an in cellulo biotechnological tool for chemical libraries screening, in order to identify inhibitors od PPIs within dehydratases heterodimers that are not essential but virulence-liked, HadBD and HadBC. The screening of an academic chemical library dedicated to PPI inhibition has been achieved, i) to establish the proof of concept of the designed screening tool and ii) to identify molecules with antituberculosis potential.
In parallel, the 3rd part of this work concerned the optimisation of an approach of affinity purification coupled to mass spectrometry in order to obtain an exhaustive characterization of FAS-II complex composition and spatial organization. This led to the identification of a FAS-II interaction network as well as the highlight of a potential novel partner protein probably responsible for the esterification step, leading to the liberation of the FAS-II synthetized product.
The analysis of the screening results provides a serious track for the discovery of PPI inhibitor molecules in accordance with the adopted anti-virulence strategy. the research for the exhaustive composition of FAS-II complex allowed the identification of potential partner enzymes, which will allow in the future to expand the developed screening tool to novel anti-virulence PPIs.
PhD Thesis committee:
- Dr. Alain Baulard, Pasteur Institute of Lille, France – Rapporteur
- Dr. Christine Carapito, The Hubert Curien Pluridisciplinary Institute, Strasbourg, France – Rapporteur
- Dr. Emmanuelle Bouveret, Pasteur Institute, Paris, France – Examiner
- Prof. Eric Clottes, Institute of Pharmacology and Structural Biology, Toulouse, France – Examiner
- Dr. Annaik Quémard, IPBS, Toulouse, France – PhD Supervisor
- Dr. Manuelle Ducoux-Petit, IPBS, Toulouse, France – PhD Co-supervisor
- Dr. Fabienne Bardou, IPBS, Toulouse, France – Invited Member